A Resounding Yes: Advancing Scientific Exploration
In the history of mathematical and scientific discovery, few figures are as enigmatic and inspiring as Alexander Grothendieck.
Berlin
Let us head back to the city of Berlin, around the year 1928. His parents were anarchist, and his journey from the internment camps of Vichy France to the very pinnacle of mathematical achievement shows a certain resilience of human spirit we get to admire in the few, the unquenchable thirst for knowledge expressed. Grothendieck became a witness of the big wars, and through struggle his deep intellectual gift blossomed. Eventually, through trial and error, and deep intellectual reflection, he figured out how to contribute through algebraic geometry ,creating a legacy extending far beyond theorems and proofs; his world of ideas encompasses an intense engagement with the ethical dimensions of scientific inquiry and a passionate advocacy for the ecosystem, oceans and animal life alike. Indeed, his early life was marked by adversity and displacement. Since his parents were Russian-Jewish , his childhood was overshadowed by the rise of Nazism, the Jews being prosecuted. His family's flight from Nazi Germany to France and their involvement in the Spanish Civil War set the stage for a chain of dramatic events, and soon he would find himself challenged by both intellectual pursuit and political activism he needed to sustain for obvious reasons. The internment of Grothendieck and his mother in the Rieucros concentration camp during World War II, coupled with the tragic death of his father in Auschwitz,did shape his perspectives on humanity, justice, and the role of intellectuals in society. Life would bring luck, apart from the obstructions he had to endure and overcome, once he managed to nullify some of these adversities, and his struggle would bear fruits, Grothendieck's passion for mathematics not only would endure but flourish.
Paris
At one point, he made an important call, leading to his admission to the seminars of the École Normale Supérieure in Paris , and this very act of his marked the beginning , of an extraordinary mathematical career. Grothendieck's ability to solve fourteen significant mathematical problems within six months, each worthy of a doctoral thesis, showcased his unparalleled genius, and nobody of his time would match. His membership in the Bourbaki group and his appointment at the Institut des Hautes Études Scientifiques (IHES) in Paris were crucial in his contributions to algebraic geometry, earning him the Fields Medal in 1966—a recognition he famously declined in protest against the Soviet treatment of dissidents. Mathematical Contributions Schemes Theory Grothendieck introduced the concept of schemes, which generalized the notion of algebraic varieties. This framework allowed for a more flexible and powerful way to study the solutions to polynomial equations, bridging the gap between algebraic geometry and number theory. In mathematical notation, an affine scheme is a pair for some ring , and a sheaf of rings called the structure sheaf. Topos theory, introduced by Grothendieck, generalizes the concept of a space and the functions between spaces, which are central to topology, to a more abstract setting that can accommodate algebraic geometry and other areas of mathematics. A topos is a category that satisfies conditions such as having all finite limits and colimits, exponentials, and a subobject classifier. An important part of his influence has been the Grothendieck-Riemann-Roch theorem, and while this is a far-reaching generalization of the classical Riemann-Roch theorem, we relate this idea to Riemann surfaces. These practical insights would lead to the creation of powerful tools for computing the dimensions of spaces of sections of line bundles on algebraic varieties. Furthermore , Grothendieck's work on étale cohomology provided new methods for advancing understanding related to the properties of general algebraic varieties. This new understanding has become essential in the study of number theory and arithmetic geometry. He would offer insights into the behaviour of solutions to polynomial equations over various fields and eventually his influence would extend beyond his specific contributions to algebraic geometry. Today these ideas have permeated various fields of mathematics, inspiring new research directions and methodologies. The concepts he introduced have become fundamental tools for mathematicians, shaping the way modern mathematics is practiced and understood. The shocking revelation in 1970 that the Institut des Hautes Études Scientifiques (IHES) was receiving funding from the Ministry of Defence marked a crucial turning point in Grothendieck's life. This revelation unfortunately, led him to resign and to reevaluate the role of science and mathematics in society. His founding of the magazine Survivre (eventually known as Survivre et Vivre) marked his full-fledged entry into green politics and activism. Grothendieck's critique of scientific research, especially its complicity in ecosystem degradation and militarization, was radical for its time. His honest call for a cessation of scientific research in his courses and lectures at the Collège de France underscored his belief in the need for a fundamental shift, in the scientific community's priorities. Now, we should note, it was in fact, in this context that Grothendieck delivered his poignant speech at CERN in 1972, a moment that crystallizes his transformation from mathematician to activist. In his important and witty address, Grothendieck carefully examined the very foundations of scientific research, urging his audience to reflect on the broader implications of their work. He argued that the pursuit of scientific knowledge for its own sake, without regard to its impact on humanity and the planet, was a path fraught with absolute peril. Challenging his somewhat surprised audience , he would push them, during his speech ,to reflect upon the broader implications of their work beyond the pursuit of knowledge for its own sake. He urged the scientists in the room to ask themselves whether their research serves a purpose contributing to humanity and the planet. Innovation
He emphasized the ethical responsibility of the minds present, for them to be aware of the potential applications of their aspirations, especially in the context of military use and ecosystem degradation. Grothendieck's speech highlighted the moral dilemmas faced by scientists when their work is used in ways that contradict their personal values or harm society. At the very core of his message hence ,Grothendieck pointed out the interconnectedness of scientific research with the survival of humanity and the health of the planet. He argued that the ecological crisis and the threat of nuclear annihilation were directly related to the advancements and applications of scientific knowledge. Needless to say, he advocated for a new approach to science—one that is ethical, responsible, and aligned with the greater good. Grothendieck envisioned a science that contributes to solving the pressing challenges of our time. We grapple with the existential threat of climate change today. Grothendieck's message is absolutely more relevant than ever. The scientific community has a critical role to play in addressing this crisis, today and tomorrow, not only through research and innovation but also by advocating for policies that protect the environment and promote sustainability. The spirit of Grothendieck's CERN speech urges all of us to question the purposes and consequences of our scientific endeavours, to ensure that they serve the interests of humanity and our mother earth. We should indeed challenge the notion that all scientific knowledge is inherently good. In the context of the troublesome climate change related issues, we notice today, this invites a critical examination of how scientific research is directed and funded. Are we prioritizing research that helps us understand and mitigate climate change, or are we still investing heavily in ignorant technologies that contribute to the problem? His idealism ansich, challenges us to rethink the societal structures that support unsustainable scientific and hi-tech advancements. There is a demand for innovation. Innovation today involves embracing vision for a transformative science that serves humanity and the planet. It means advocating for research that prioritizes the common good, fostering ethical considerations in scientific endeavours, and recognizing the value of diverse forms of knowledge in addressing the climate crisis. We can inspire others, providing our thinking is critical and indeed, providing we maintain a certain reflective approach to science. Our pursuit of knowledge contributes to a hi-tech and equitable future for all. Alexander Grothendieck's legacy serves as a light flickering within the night, for all who believe in the transformative power of science to create a better world. His life's work and his activism remind us of scientific exploration, in such a way, when guided by a commitment to social and environmental justice, can be a complete force for good. We can confront the challenges of climate change and other global crises, because we are capable, and since we are capable, abandoning passive habits will allow us to advance scientific exploration in the spirit of Grothendieck's work. A resounding yes to a science that is ethical, responsible, and dedicated to the well-being of all! Sources: Grothendieck, A. (1972). Speech at CERN. Grothendieck, A. (1988). Refusal of the Crafoord Prize. Pessis, C. (2015). Alexander Grothendieck: Tribute to the Deceased. CQFD Monthly, No. 128. "The ecological crisis and the threat of nuclear annihilation were directly related to the advancements of scientific knowledge."
- Grothendieck, 1972
Decyphering Grothendieck his notes.
Cubicles
Alexander Grothendieck his scribbles were a mystery at first. He passed not that long ago and, someone has to look into them, someone has to do it. After his passing, he left behind a vast collection of these notes, scribbles, and manuscripts and drawings, but what do they contain? There are some dozens of pages. The prospect of exploring these has felt daunting at first but eventually i thought i could cope so I decided to take the plunge. I've begun the process of trying to decipher some of these posthumous notes. By now i can browse through the pages, understanding a good bulk of them, i suspect eventually i might be able to translate most of them if not all. It started small, focusing on just the initial few pages, the first scribbles I could access. However, wading into these first few pages has already shifted my perspective. My initial impression is that these specific notes weren't necessarily left behind with the direct intention of "advancing science" in the way a polished paper or a finished theorem might (his habit throughout his carreer). They feel different. They seem much more like intermediate structures, snapshots of a fine mind at work, building blocks within his own intricate thought processes rather than finished edifices intended for immediate public consumption. Does this make them less valuable? Seeing them as part of a process doesn't diminish their importance in my eyes. There's immense value in witnessing (even retrospectively and partially) how such a keen intellect worked through problems, the scaffolding he built before reaching his known peaks. They deserve appreciation for what they are: intimate glimpses into a remarkable intellectual journey. Based on these initial attempts, I feel optimistic. The language, though dense and characteristic, feels approachable. I have a growing confidence that, given time, I could translate the rest too. Interestingly, this dive into pure, abstract mathematics runs parallel to my own ongoing work. I've also made some small, but steady, progress in my photonics research recently. The ultimate goal there remains crystallizing an idea, perhaps pointing out a photonic law more properly, or at least illuminating its potential more clearly. And perhaps these two threads – the historical deciphering and the future-facing physics – aren't entirely separate. I have this strange, persistent impression that these deep mathematical structures, the kind Grothendieck explored, will somehow loop back into my life and work, perhaps even geographically. It's a bit whimsical, but I can almost picture myself puzzling over these notes, or finally formulating that photonic principle, while living amidst the beauty of the Philippines, since i have been enjoying the calm of its coral beaches, and building my business there along my spouse. For now, though, it's one step at a time. One page of Grothendieck deciphered, one small advancement in photonics. It's a journey, and I'm excited to see where both paths lead. The first i will cover here is a cube.
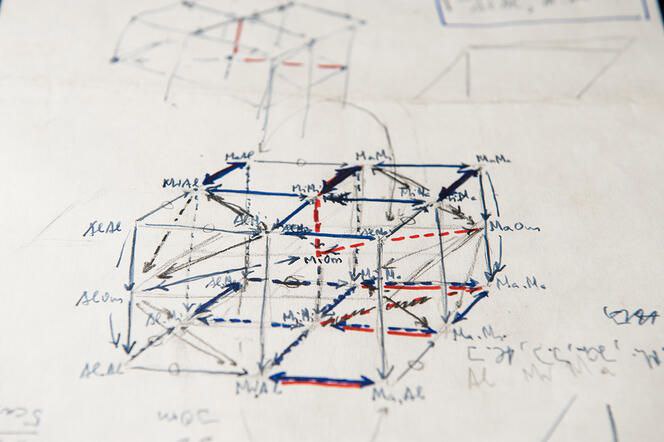
Grothendieck was exploring dimensions here related to category theory. The cube represent several nodes, and morphisms (he used arrows to draw these), the morphisms he created between them. The cube structure was designed to organize the morphism. The framework is a scaffold, you can see the vertices and edges drawn, forming the outline and a fainter simpler cube is visible atop. The digraph (atop) Is there partial to sustain vertices (think of them as nodes) and they point along the edges and within the volume (faces) hence serving as true nodes. He wrote down some maths near each node, using M as his point of reference: Here M is meant to point out states and variable within the system he was modelling. The system is an Arc, because the nodes connect with lines by arrows representing directed edges. They are indicate relationships, helping him draw the transitions and hence transformations (or implications) between those nodes. So you could say he was drawing multiple dimensions ,since he was interested in certain dimensions his cube would clarify for him: the cube axes would point them out. Note that there are multiple edge types, hence he used different colors for each and he changed his arrows too (thick black, or thin black and dashed blue and solid red) These represent the categories (or relationships if you may) and paths within his structure. Keep staring and you will notice the Arc. Perhaps later on i will cover more of his notes.
Regarding my own work, i do not have much to brag about right now. There are certain obstructions that remain unresolved for now. The photon's motion occurs only within boundaries permitted by dimensionally sustainable adjustments in my work and i need to use functors to solve part of the problem. So far i was able to draw the spacetime manifold, since it must adapt its structure in infinitesimal steps to maintain the photon’s categorical invariance. I am using Mirzakhani her systems, to create my approach. (the rate of change of the photon soul with respect to the system's evolution is identically zero) I have a difficult time solving this problem, but i think i will eventually, providing i invest enough time and energy.
It is not the photon's energy, mass, or spin alone that enforces this behavior — it is the preservation of its categorical soul structure. I suspect i could create some sort of diagram to point out photonic evolution, but as of now, it is unclear to me how.
So far I did manage to draw from Langlands to bridge number theory, modular forms, and quantum field structures.Light is not merely a physical phenomenon — it is a manifestation of the deep arithmetic-geometric structure of reality itself. Where will it all lead if i succeed? A deeper understanding of gas? Improved research of time crystals? Fusion energy applications? Light persists not because nature restricts herself, but because nature seeks to sustain herself. My work argues the separation of light from matter is not mechanical, but categorical: it emerges from the preservation of an underlying soul structure sustained across manifold evolutions. Wish me luck. From Neurons to Networks: Envisioning AGI
AGI
Artificial General Intelligence (AGI) has been recently in the press. Most people have heard the term AI; however, AGI is where we are heading. AGI represents a great kind of progress regarding artificial intelligence research, since we are aiming to create machines capable of performing any intellectual task that a human can. While current AI systems excel in specific domains, AGI aspires to achieve a level of cognitive flexibility and understanding akin to human intelligence. Understanding the fundamental building blocks of biological intelligence—neurons—to envisioning the complex networks that could underpin AGI, is part of this process, because through the lens of molecular biology AI can be understood more thoroughly. The molecular reality of the brain consists partially of neurons, the Principal Units Neurons are the principal cells responsible for processing and transmitting information within the brain. When we investigate deeper, we notice each neuron consists of a cell body (soma), dendrites, and an axon. We know these dendrites are the ones receiving signals from other neurons, while the axon transmits signals to other neurons. Along the synapse, a specialized junction between neurons, the transmission of these signals takes place through neurotransmitters and hence we observe at the molecular level, how neurons communicate through a complex interplay of electrical and chemical signals. These lessons can be applied to aid us in our dreams, developing AI. Ion channels, neurotransmitter receptors, and various signalling molecules play crucial roles in the process and understanding these molecular mechanisms is essential for replicating neural functions in artificial systems.
Neural networks
Let us consider neural networks. Biological Neural Networks located in the brain, consist of neurons because they relate to cognition. These neuronal structures are organized into sophisticated networks that facilitate complex cognitive functions since these networks are characterized by their connectivity patterns, synaptic strengths, and plasticity. Synapses have the most curious ability to strengthen or weaken over time, and their ability is a key mechanism underlying learning and memory. The computational side, artificial neural networks (ANNs) express our model. We design them to mimic the brain's structure and function. The vast potential of these ANNs is obvious, and they consist of layers of interconnected nodes (analogous to neurons) that process information through weighted connections (analogous to synapses). Training these networks involves adjusting the weights based on input data, a process akin to synaptic plasticity. Extensive advancements have been achieved allowing for commercial models such as the models OpenAI has released, and while current AI systems are constrained by their narrow focus and lack of generalization, they do excel in specific tasks but struggle with tasks outside their training data. This limitation arises from the rigid and predefined architectures of existing AI models. Through a Molecular Lens achieve AGI will be no doubt achieved more rapidly since researchers are exploring the molecular underpinnings of intelligence. By our efforts studying the molecular biology of neurons and neural networks, we aim to uncover principles that can be applied to artificial systems. Such challenging, daunting task involves integrating knowledge from neuroscience, molecular biology, and computer science to create more flexible and adaptive AI models. Next-generation molecular computing, which leverages the principles of molecular biology to perform computations allow us to reflect and finetune our products. Molecular computing systems use molecules, (including DNA and proteins), in order to store first, and second process certain data. These systems offer the potential for massive parallelism and energy efficiency, and hence these are key attributes for sustaining AGI. Neuromorphic engineering is another approach that seeks to emulate the brain's architecture and functionality in hardware. By designing circuits that mimic neural processes at the molecular level, researchers aim to create more efficient and powerful AI systems. Neuromorphic chips, for example, use spiking neural networks to replicate the brain's communication patterns. Molecular computing
There are several aspects of neurology currently of interest for the development of advanced large language models, especially Neurogenesis (Neurogenesis being the process of generating new neurons), along with synaptic plasticity, are fundamental to learning and memory and it is no surprise recent studies have shown that neurogenesis continues into adulthood, especially in the hippocampus, a region associated with memory formation. Understanding these processes at a molecular level can provide insights into creating more adaptive and resilient AI systems. At the cellular level, Glial Cells including the astrocytes and oligodendrocytes, play crucial roles in supporting neurons. Astrocytes regulate neurotransmitter levels and maintain the crucial blood-brain barrier (in turn protecting the brain), while oligodendrocytes insulate axons with myelin. An interesting remark to make is how Incorporating the functions of glial cells into AI models could indeed enhance their efficiency and robustness. Neurotransmitters such as dopamine, serotonin, and glutamate are essential for modulating neural activity. (Dopamine, being involved in reward processing and motivation) By modelling neurotransmitter dynamics, AI systems will no doubt improve, as we design them to be better, they will mimic human decision-making and emotional responses. Brain-Computer Interfaces (BCIs) enable direct communication between the brain and external devices. These peculiar advances in BCI technology have the potential to enhance human-AI interaction, allowing for more intuitive and effortless integration of AI into daily life. Because AI is important for molecular medicine, the medical community will support and contribute the development of AGI and understanding the molecular basis of neural signalling can improve the development of BCIs. One productive approach to help develop AI is through the study of Artificial Neural Networks (ANNs), since these have experienced significant advancements, especially with the advent of deep learning techniques. These networks, composed of multiple layers, can learn complex patterns from large datasets. The most useful language right now to approach AGI is Python, and Python proves to be both simple and complex though we will likely end up developing more specialized languages. Python has become a standard tool for developing and training ANNs due to its extensive libraries such as TensorFlow and PyTorch. The Supercomputers we have developed today, will progress into AI Supercomputers and related hardware play a critical role in advancing AI research. Since they provide the computational power necessary to train large-scale neural networks and simulate complex biological processes, they use plenty of energy, hence the switch towards using more green setups is of essence. Recently google has released AlphaFold 3, the latest iteration of their project called DeepMind, and AlphaFold 3 is all about DeepMind's protein structure prediction capabilities, through this exact prediction model, google has revolutionized drug discovery. By accurately predicting protein structures, AlphaFold 3 enables researchers to understand disease mechanisms and identify potential drug targets more effective. Google their breakthrough does demonstrate the renewed potential of AI in transforming biomedical research. We are now capable of analysing vast amounts of biological data, we can identify novel drug candidates, and optimize our drug design process. Machine learning algorithms can predict the efficacy and safety of new drugs, significantly reducing the time and cost associated with standard drug development processes. By understanding and replicating the fundamental principles of biological intelligence, we can envision a future where machines possess the cognitive flexibility and understanding of humans. This endeavour not only advances our hi-tech capabilities but also deepens our understanding of the nature of intelligence itself. BNNs interconnected with robots can exhibit clever behaviours such as learning and memory, which are crucial for developing AGI systems. Our normalization Strategies involves parallels between artificial and biological neural networks, which are essential for stabilizing network activity and enhancing learning efficiency, hence our vision regarding AGI has proven to be somewhat functional. The development of AGI necessitates addressing certain societal implications, including the impact on employment, privacy, and security. For example, the use of high-density CMOS microelectrode arrays in engineered biological neural networks can significantly enhance the spatiotemporal resolution, aiding in the development of more sophisticated AI models, and since these models are rapidly evolving, we need to control them while we allow them to empower our research. Drug Discovery AI technologies, especially AlphaFold 3, have revolutionized drug discovery by accurately predicting protein structures, only contributing to the field of medicine, demonstrating the transformative potential of AI in biomedical research. While a variety of open source LLM’s have been released so far, privacy and security regulation will become more of essence in the future. A new hi-tech saga begins.